Latest Publications
How to beat the heat in cities through urban climate modelling
Jan Carmeliet is an expert on urban climate and heat wave mitigation. He is professor at the Chair of Building Physics at the Departement of Mechanical and Process Engineering of ETH Zurich. Dominique Derome specializes in building and urban physics aiming for a more resilient built environment. She is professor at the Université de Sherbrooke, Canada, and holds a Canada Research Chair tier I in Multiscale Building Physics. As experts in the field, the two researchers were asked to write an exclusive article for the journal Nature Review Physics to explain what factors make cities turn hot, and what can be done to cool them.
The goal of the publication is to help the physics community contribute to sustainable goals. The comments paper was published on 22 November 2023 and is part of a collection of articles on the subject of physics and sustainability that the journal published this year.
Article: external page https://rdcu.be/drKeY
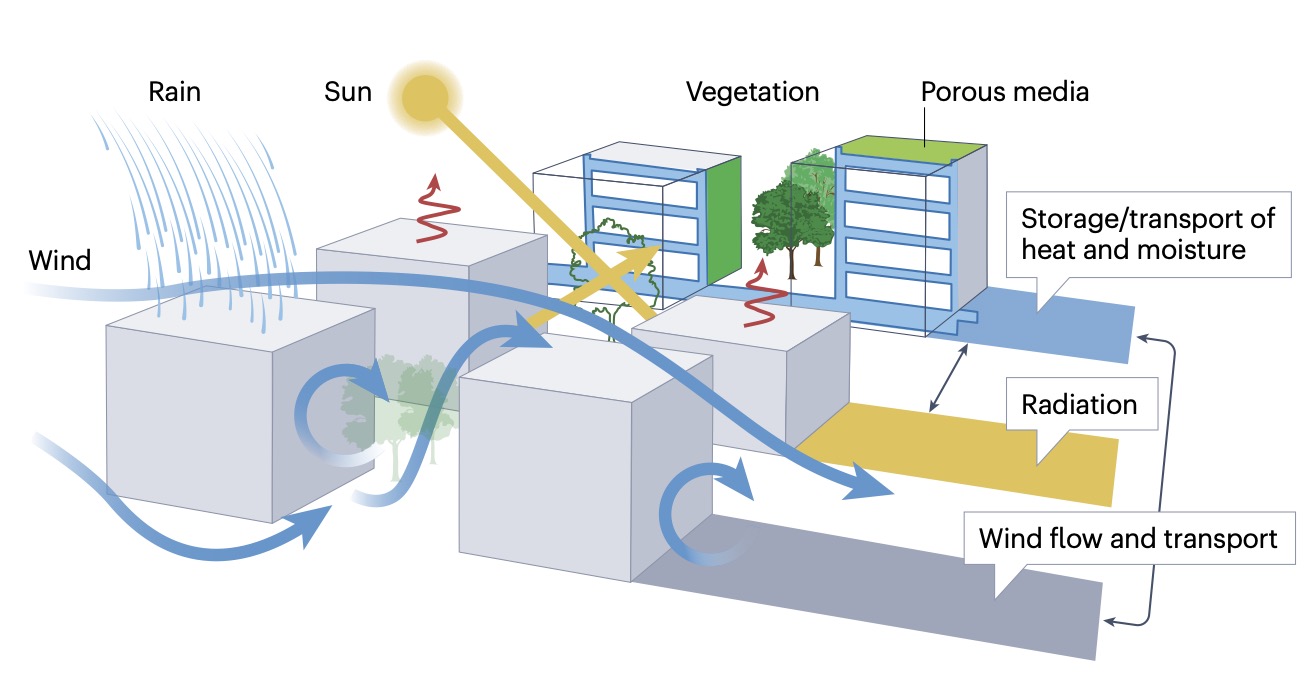
Cool surfaces against urban heat islands
The use of permeable and reflective pavements as a potential strategy for urban heat island mitigation
The urban heat island (UHI) effect rises temperature in cities, increases the energy demand for cooling and has negative impacts on thermal comfort and health. This phenomenon is related to the positive thermal balance created over urban environment, where open land and vegetation are typically replaced by buildings, roads and other infrastructure, causing an increase in heat gains and a decrease in thermal losses. Past research shows that the choice of urban materials strongly influences the local urban temperatures through their role in the absorption, transport and storage of heat and moisture. However, a large fraction of urban areas consists of impermeable and/or dark-colored surfaces, such as conventional asphalt and concrete, which adversely contribute to the UHI effect. Urban surfaces that enhance evaporative cooling with porous materials, or that use reflective materials can help with the mitigation of the UHI effect by reducing the outside air temperature, especially during extreme heat wave events.
The cooling potential of evaporative cooling depends on a combination of factors such as moisture permeability, moisture capacity of materials and available moisture content. Furthermore, the duration of evaporative cooling is also influenced by external environmental conditions, such as radiation, local air temperature, relative humidity and wind speed. Therefore, in order to assess the ability of reflective and permeable pavements to be used as possible mitigation measures for local urban heat islands, it is essential to be able to predict the local scale microclimate as a whole.
Dr. Andrea Ferrari, an ETHZ postdoctoral researcher, together with Dr. Aytaç Kubilay from Empa, developed and applied an urban microclimate model to investigate the cooling potential of surfaces with improved hydraulic and thermal properties as a promising counter-measure to heat islands at local scale. The microclimate model includes two subdomains: the air domain and the domain consisting of the porous materials. Computational fluid dynamics (CFD) is used for solving the turbulent air, heat and moisture flow in the air domain around buildings. Spatial and temporal variations of local temperature, humidity and wind speed are, then, coupled with three additional submodels: 1) a model for heat and moisture (HAM) transport in porous urban materials, 2) a radiative exchange model, determining the net solar and longwave radiation on each urban surface and 3) a wind-driven rain model, determine the wetting flux on each surface during a rain event. Evaporating cooling is efficient as long as liquid transport inside the material can provide sufficient liquid water to the drying surface, so that the highest reduction in surface temperature occurs. The numerical model allows for the prediction of detailed moisture distribution in urban surfaces, making it possible to evaluate the impact of different types of urban materials on the urban microclimate under both dry and wet conditions.
The aim of the study is to analyze and understand the role that evaporative cooling and reflective materials can play in mitigating heat islands at local scale. The study focuses on a street canyon with different multilayer pavement solutions by optimizing their moisture transport properties and layering structure in order to achieve the highest evaporative cooling effect. Afterwards, pedestrian thermal comfort, based on mean radiant temperature and local variables such as air temperature, relative humidity and wind speed, is compared to evaluate the effectiveness of different configurations of pavements.
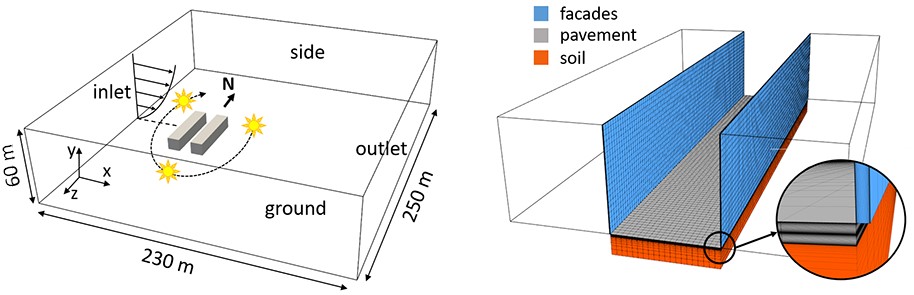
The results show that pavement layers with coarse and fine pores can be efficiently combined in order to collect a sufficient amount of water when wet and promote capillary transport at the surface during drying. Porous pavements composed of layers with fine layers at top and coarse layers at bottom are found to enhance evaporative cooling by trapping water close to the surface. As a result, a lower surface temperature by up to 20°C is achieved compared to conventional concrete. Such an impact is sufficient to decrease thermal stress level at local scale in the street canyon. The use of reflective surfaces also decreases the surface temperatures significantly, but their biggest impact seems to be when used in combination with permeable pavements, as this leads to a longer duration of evaporative cooling effect.
Developing urban surfaces specifically designed to mitigate heat stress is of high scientific as well as social and economic relevance. In particular, new findings and guidelines for cool pavements and green surfaces can provide a decision support platform for local governments, communities and urban-planners. This work is published in the Urban Climate journal. external page Link
Machine Learning Reveals Frictional Failure
Research in Collaboration with LANL
Spatial and temporal forecasts of earthquakes are among the biggest open challenges in Earth Sciences. A major difficulty in studying earthquakes is the limited possibility to investigate seismic events at the point of its origin, deep in the Earth. Real earthquakes can only be analyzed by seismometers far away from the exact point where the rupture takes place. To get better insights in the genesis of earthquakes, scientists mainly rely on two approaches i.e. building a scaled model in laboratory conditions to replicate the behavior of shearing and colliding tectonic plates or building a computational model for simulating the behavior of fault damage zone under shear.
In our recent paper in external page Geophysical Research Letters, we have employed a computational approach using Discrete Element Modelling to simulate a granular fault gouge that is slowly sheared between two plates. This configuration is shown to replicate the characteristic stick-slip dynamics that is suggested to be the main mechanism for earthquakes nucleation and rupture processes. Stick-slip dynamics describes a periodic process of building up strain energy in the system, which is suddenly released in a slip, as an earthquake.
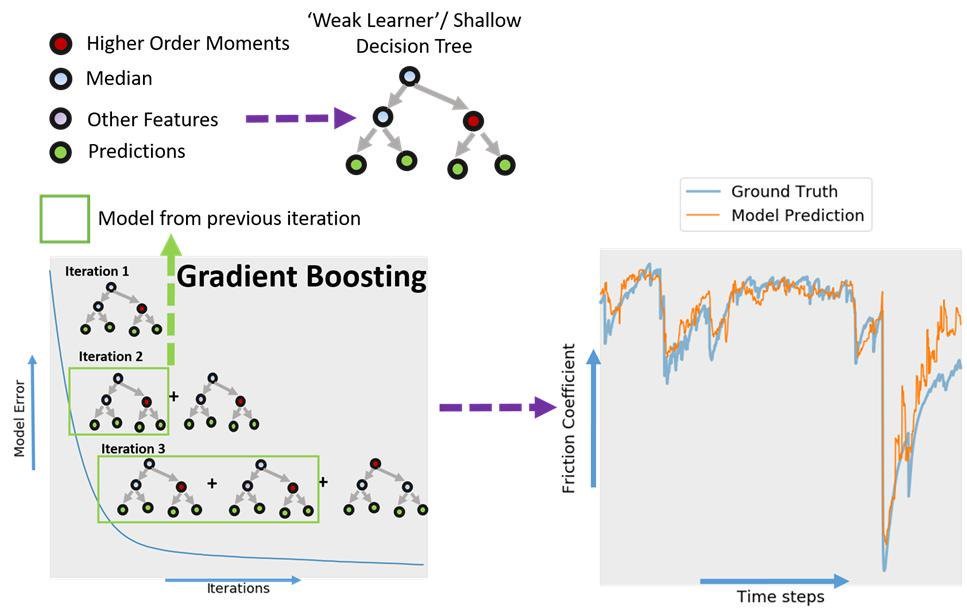
In collaboration with researchers from Los Alamos National Laboratory (LANL), we combined simulations and machine learning techniques to find new ways of predicting stick-slip cycles in the temporal domain of these simulations. In our paper, we use the statistical properties of the velocity signals from all particles in the system during a short moving time window as an input for a gradient boosted decision tree model. We fit this velocity signals to the macroscopic level of friction in the system, which represents the stick-slip cycles in the sheared granular model. Results of decision trees have the advantage of being physically interpretable, compared to much more popular deep learning techniques. We use boosted trees to increase the quality of the fit. As this is a supervised machine learning approach, we split the time series of the model into a training set containing the first 80% of the simulated data and a testing set with the remaining 20% of the time series to evaluate the performance of the fitted model. We also find the important features in the model to get physical insights of the system. To this end, we use a recent method called Shapely Additive Explanations values developed based on game theory.
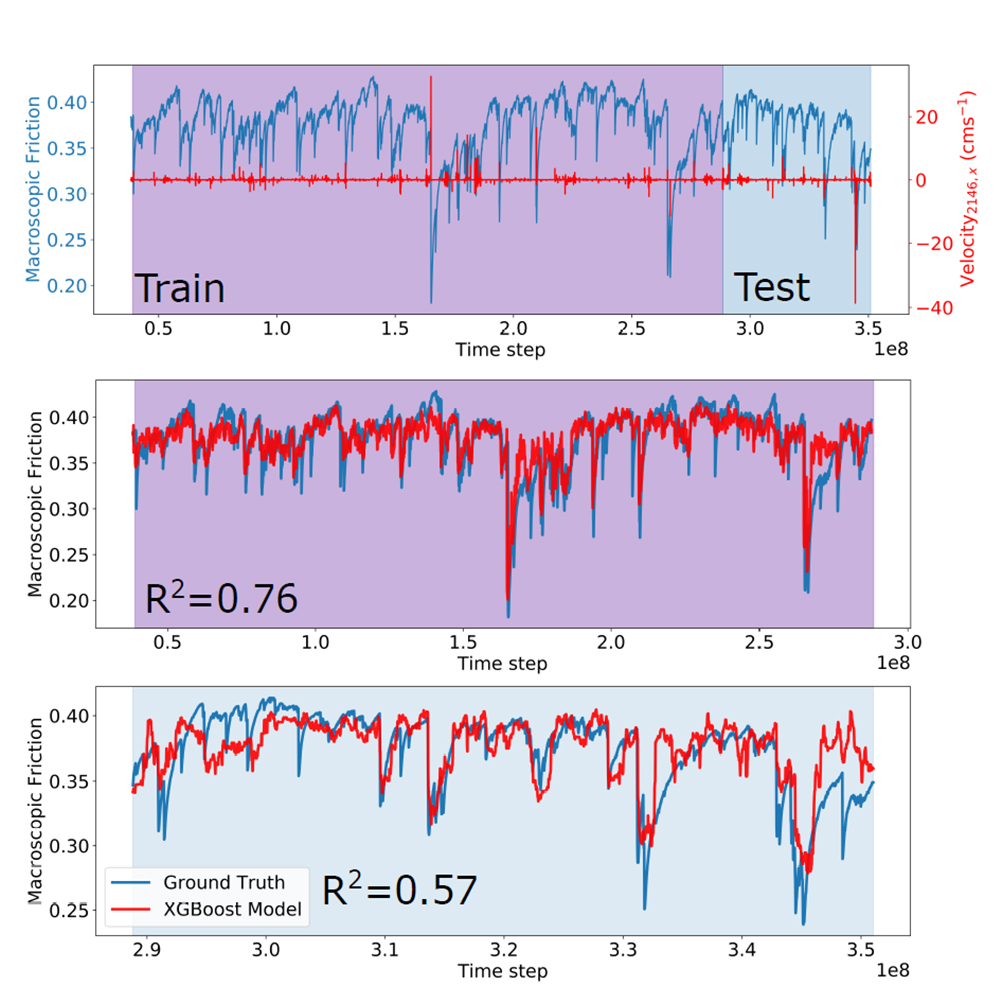
Our paper shows the potential of combining simulation and machine learning in Earth Sciences and in general in characterization of stress and deformation for better understanding of the genesis of earthquakes and other types of frictional failure. The similarities between our model and field‐based observations from real faults suggest that an extension of our approach may estimate the friction of geological faults that can lead to prediction of real earthquakes. We will continue to work with our simulation data by using different techniques to improve the understanding of macro- and microscopic behavior of fault systems. For more information, please contact Omid Dorostkar () or Dominik Strebel ().
Origin of Sorption Hysteresis in Wood: our research published in Nature Communications.
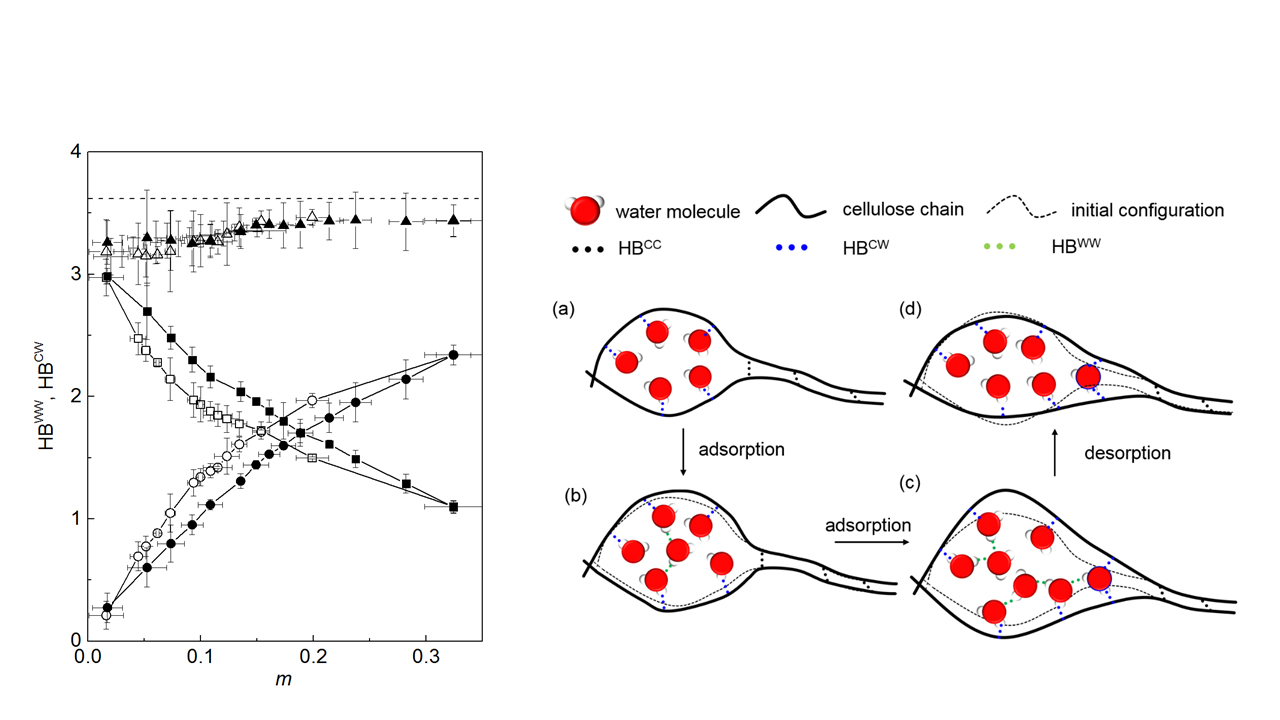
A tree relies on the strength of its building material to stand up. Being moisture sensitive, how can it cope with the continuous variations in relative humidity? How can wood, used in buildings, furniture or supporting paintings, do the same? This is due to hysteresis, a passive mechanism embedded in its polymeric nanostructure that allows wood to hold its moisture under varying relative humidity. This mechanism also allows important wooden artefacts, like the painting of the Mona Lisa, from cracking. Hysteresis has been observed since long, now it is understood.
We have used molecular dynamics to look into the voyage of water molecules through amorphous cellulose and elucidated the mechanisms that lead to moisture hysteresis, the capacity of a material to hold almost the same moisture content although exposed to varying relative humidity, as reported in a recent Nature Communication publication. Water is captured at sorption sites during increasing relative humidity, called adsorption and remains preferentially at these sites when the relative humidity decreases, called desorption. Hysteresis allows plant cell walls to keep their water while undergoing important sweeps of relative humidity. This intriguing capacity of wood and other plant-derived materials is known for centuries, but its origin, although hypothesized, was never explained.
In molecular dynamics simulations, the water molecules are let to roam around amorphous cellulose, which are chains of glucose, a main building block to make the cell wall of plants. Hydrogen bonds are used to inform the voyage and state of the water molecules: is the water molecule free or bonded to a hydroxyl site along the polymer chains. Starting from a dry bundle of cellulose chains, water is added bit by bit. We could see that, in adsorption, due the unravelling of the bundles of cellulose chains, more and more sorption sites become available for the water molecules. When water molecules are again removed at decreasing relative humidity, they remain stuck in the deformed entanglement of polymer chains, and hysteresis appears.
Mingyang Chen, ETHZ PhD student, understood that, to study appropriately this problem, a new step in atomistic simulation was needed and successfully developed a new method where molecular dynamics simulations allowing the movement of the molecules and deformation of the material are coupled with grand canonical Monte Carlo simulation where water molecules are randomly added to reach a certain chemical potential, in layman terms the relative humidity. This combination of methods allows to scrutinize the system and its configuration in adsorption and in desorption.
Such mechanism allows the same material to become softer when wet and strong when dry. This allows in trees that the same cell in its early live, when responsible for transporting water, is soft, while it becomes stronger several growth rings later within the tree trunk. Then, this cell contributes to the strength and stiffness of the tree. When undergoing daily variations in relative humidity, little changes in moisture content occur due to hysteresis, which results in less swelling and shrinkage, thus protecting the material from cracking under fatigue loading.
Museum conservators have known since long that by minimizing the variation in relative humidity of their environment, they protect their artefacts from moisture damage. Now they understand down to the molecular scale what is going on in their collections.
Read the full article external page here.
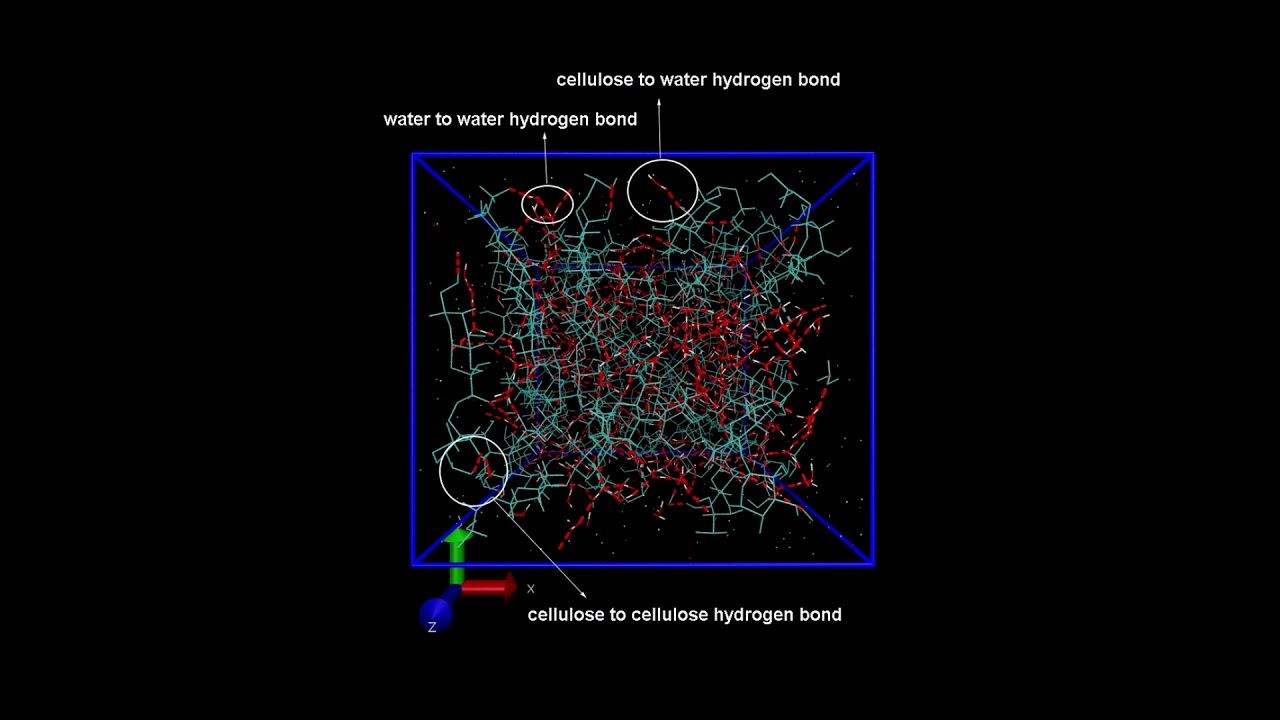